Sunday, May 30, 2010
Up and down
"LHC" seems the most obvious answer -- test the least-understood sector of the theory (the breaking of electroweak symmetry) by trying to produce the missing piece of the Standard Model (the Higgs boson) and/or hopefully something else, and unexpected. We all hope to be in Rabi's position, who exclaimed "who ordered that ?", when the muon was discovered and did not fit into anybody's plans. So should we just wait for the LHC to give all the answers ?
Fortunately not. Jester's posts show at least one place where things are moving fast, if not always in the direction of your dream -- flavour physics, i.e. the electroweak transitions under which a quark or a lepton changes from one type to another. Obviously, this is not an easy game. Quarks are subjected to strong interaction -- and thus confined into hadrons, so that you never actually observe the decay of a naked quark into another one, but rather that of a meson to one or several others. The computation of such processes is a theorist's nightmare, involving hadronic quantities that are very hard to estimate and possibly hiding faint contributions from New Physics. Leptonic processes are much cleaner, and provide signals of New Physics almost completely free of Standard Model backgrounds, like $\mu\to e\gamma$. But it is a "hit or miss" game : you need New Physics to make such processes frequent enough so that you can detect them... but you have no clue if this will be indeed the case.
The power of flavour physics lies in its sensitivity to virtual effects : by measuring accurately this process, you are sensitive to quantum corrections induced by particles/interactions at much higher energy scales. The past history has proved that it was not only theorists' daydreaming : the non-observation of $K_L\to\mu\mu$ led to the introduction of charm, the violation of the CP symmetry in the kaon system suggested the existence of a third generation of quarks and leptons, and neutrino oscillations were the first hint of physics beyond the Standard Model with the need for right-handed neutrinos. Moreover, despite our difficulties with the strong interactions, we can make (sometimes) quantitative statements : for instance, the upper limits on the difference of masses in the $B\bar{B}$ system showed that the top quark had to be much heavier than previously thought...
The present is a reflection of the past, as the philosophers say. Flavour physics keeps being a stringent constraint on many models of new physics, since they often predict transitions at much higher rates than observed. $\epsilon_K$, $b\to s\gamma$, electric dipole moments and so on have proved stubborn killers for unsuspecting theories for what lies beyond the Standard Model. They are indeed so powerful that for most theories, they push the scale for New Physics much above 1 TeV to make their impact negligible on the processes currently observed... Eh but wait a minute ! This is not what we want -- we want New Physics at the TeV scale !
Fortunately, you can find specific scenarios (such as Minimal Flavour Violation) to accomodate both the data from flavour physics and our LHC expectations. But it would be far more natural (historically speaking) and exciting (scientifically speaking) to see hints of new physics already in some flavour processes, before actually producing new particles at LHC responsible for these deviations. Hence all the recent discussions on the CDF/D0 results, taking back from one hand (CDF $\phi_s$) what they gave us from the other (D0 $A_{SL}$). That is the fundamental problem of flavour physics: since you do not observe new particles, you must spend your time on assessing how much your process has drifted from the Standard Model expectation. And it is a cruel game. Among the many deviations from the Standard Model observed in flavour physics, the majority stays in the grey area between 2 and $3\sigma$ before fading slowly into 1 $\sigma$ oblivion after a more careful scrutiny, both from experimentalists and theorists... Who is still excited by the $b\to s\bar{s}s$ processes, which used to deviate at 3 $\sigma$ from the Standard Model expectations and are now in perfect agreement with it ?
Are the recent results from CDF and D0 two different points in these Russian hills, or will one of these anomalies stay with us to finally be confirmed as a real effect ? Only time -- and experiment ! -- will tell.
Saturday, May 29, 2010
Telescope transport now leaving from gate A...
Out on the job market in the real world, physicists are popular people. They can get almost any job because, well, they can do almost any job. They are good at maths, computing, logic, solution-oriented and creative thinking. They are used to working in teams, in different languages, with different cultures, so when physicists decide not to stay in physics, they might end up as investment bankers, software developers, patent lawyers, safety specialists, teachers, consultants. And probably many other professions that I’m not even aware of.
But it’s not only in life after physics that their versatility is useful. As particle physicist you are as much a brainy boffin as you are a technician with screwdriver in hand, or a manager, or an accountant – and sometimes you can be a truck driver. Tomorrow morning at 8:30 a special kind of van will leave the German lab DESY and go to CERN to deliver a beam telescope. At the wheel: coordinator and physicist (and blogger) Ingrid Gregor…
The beam telescope is about as versatile as the people who use it. You need it to check whether what you think you see with your detector really is there, a sort of cross-check mechanism, only with lots of added value. In labs around the world, scores of physicists are already working on new generations of particle detectors – like the ones busy taking data at the LHC at CERN right now. These need to be tested, and the best way to test a particle detector is to put in a beam of particles, a test beam. There are test beams at all the major labs around the world, including DESY and CERN – particles circulating in the accelerators are directed to separate areas where many smallish experimental stations receive them. Different accelerator deliver different particles, and depending on the type of tests you want to make some are more useful than others.

The beam telescope – the product of the European ‘EUDET’ project that coordinates detector development infrastructures – gets into the ring with the test detectors (all sorts of different kinds, by the way) and tests their precision. A highly precise tool itself, its six boards, fitted with silicon pixel sensors, can measure the beam particle tracks to a precision of three micrometres, while another device, typically pixel or strip detectors, can be positioned in the middle to be studied. (I stole this sentence from a NewsLine story in case you’d like to read up on the telescope). It goes to CERN for a round of shifts with ATLAS detector upgrades and has an extremely full agenda at CERN until the end of November, when Ingrid gets back into the telescope truck and hauls it back to DESY.
And now the best bit: I’ll be part of the delivery! I’ll be driving down with Ingrid, Volker the technician, EUDET the telescope and a stack of freshly made CDs…it’s a twelve-hour trip after all. You’ll be hearing more of it later!
Important update from CDF
Disappointingly for most theorists, another Tevatron's experiment CDF recently presented an update that reverses that trend. CDF repeated the measurement of $B_s \to J/\psi \phi$ on a larger data sample of 5.2 inverse femtobarn, that is with 2 times larger statistics than in the previous measurement. The new result is consistent with the standard model at the 0.8 sigma level:

Wednesday, May 26, 2010
What should we expect from LHC?
In the next few months there will be three main conferences where physics results from the LHC experiments will be presented: the nearest one is Physics At LHC, that will take place at Desy in Germany the second week of June; the second one is, erm... you know... ICHEP; the third one is the Hadron Collider Physics Symposium in Toronto, at the end of August. The kind of results one might expect to be presented at each of these conferences is rather different. The LHC is in fact steadily delivering proton-proton collisions at 7 TeV: the farther in time the conference, the more integrated luminosity the experiments will be able to use for their analyzes.
Could we try to guess what is likely to be shown at ICHEP by ATLAS and CMS? Well, it's definitively not an easy prediction: even assuming a perfect efficiency of the two experiments in collecting the data and analyzing it, the LHC beam conditions are improving every day, and the exploitable integrated luminosity at - let's say - mid July can largely vary.
Let's then try first a different exercise: which results are more likely to be seen at a conference as a function of the integrated luminosity collected at 7 TeV, from the small amount we already know as been secured by the experiments to the 1 fb-1 promised by the machine for the end of the 2010-2011 running? Warning: what follows is a very approximate list, I might have missed important signals here and there, and my judgment is certainly biased by my ATLAS experience. Here's what we'll get (or what we already got):
- 10-100 μb-1: millions of charged pions to happily redo the charged multiplicity analysis published with the 900 GeV data collected in 2009; a few tens of $J/\psi \to \mu \mu$, a few jets here and there. Any resonance that can be spot using the tracker system (like K's and $\Lambda$'s) has been been seen at this point; signal from $\pi^0$ and $\eta$ decaying in photons pairs is found and well isolated.
- 100-1000 μb-1: any hint of a $J/\psi \to \mu \mu$ peak should now be clearly visible;
- 1-10 nb-1: more jets. And of course more jets-related measurements.
- 10-100 nb-1: a few tens of W begins to appears in the data. The lucky ones might have seen a few Z bosons. A first observation of prompt inclusive electrons should be at reach at this point.
- 100-1000 nb-1: more and more jets. The first inclusive muon measurements should be feasible. Signal from prompt photons should have been isolated.
- 1-10 pb-1: at this point ATLAS and CMS should have secured enough W and Z to dare to attempt a first cross-section measurement. They might be able to pretend to have seen the top quark.
- 10-100 pb-1: first B-physics related measurements. Something could already be said about some exotic scenarios, and some SUSY points.
- 100-1000 pb-1: at this point, one could even optimistically hope in some timid news about the Higgs boson (exclusion), at least where the sensitivity is higher.
Of things to come
Monday, May 24, 2010
A perfectly workable unit
Wednesday, May 19, 2010
And CMS, in the meantime...
This morning, after months of work, finally a paper by the CMS collaboration sees the light. Or should I say the pre-light, since the paper has been sent to the Cornell Arxiv, and to Physical Review Letters, but it is so far only a pre-print: the PRL reviewers will need to approve it for publication. Given the excruciatingly long and painful internal review process that the paper has withstood within CMS, by about 5000 eyes (or 2500 pairs if you prefer), I would say there is not a chance that the paper does not pass the standards of PRL now. But maybe it is better to be cautious, so - pre-light!
The paper reports on a measurement of Bose-Einstein correlations between pairs of charged pions recorded by CMS in its early runs at 0.9 and 2.36 TeV of center-of-mass energy. Nothing too exciting, but indeed a nice clean new measurement from data which allows little else at this stage, due to the small luminosity collected by the LHC this far.
I was personally involved in the analysis of the data for this result, so I quite well know how painful it was to produce the paper. But it has left today, so it is time to cheer up. In the meantime, CMS is working at dozens of other publications, which are expected in time for ICHEP. Paris will be brimming with new LHC results, I am sure. Another reason to look forward to it, besides the ephemeral albeit exciting news from the DZERO detector!
Monday, May 17, 2010
New Physics Claim from D0!
The measurement in question concerns CP violation in B-meson systems, that is quark-antiquark bound states containing one b quark. Neutral B-mesons can oscillate into its own antiparticles and the oscillation probability can violate CP (much as it happens with kaons, although the numbers and the observables are different). There are two classes of neutral B-mesons: Bd and its antiparticle Bd where one bottom quark (antiquark) marries one down antiquark (quark), and Bs, Bs with the down quark replaced by the strange quark. Both these classes are routinely produced Tevatron's proton-antiproton collisions roughly in fifty-fifty proprtions, unlike in B-factories where mostly Bd Bd have been produced. Thus, the Tevatron provides us with complementary information about CP violation in nature.
There are many final states where one can study B-mesons (far too many, that's why B-physics gives stomach contractions). The D0 collaboration focused on the final states with 2 muons of the same sign. This final state can arise in the following situation. A collision produces a b \bar b quark pair which hadronizes to B and B mesons. Bottom quarks can decay via charged currents (with virtual W boson), and one possible decay channel is b -> c μ- νμ. Thanks to this channel, the B meson sometimes (with roughly 10 percent probability) decays to a negatively charged muon, B -> μ- X, and analogously, the B meson can decay to a positively charged antimuon. However, due to BB oscillations B-mesons can also decay to a "wrong sign" muon: B -> μ+ X, B -> μ- X. Thus oscillation allow the BB pair to decay into two same sign muons a fraction of the times.

Now, in the presence of CP violation the B->B and B->B oscillation processes occur with different probabilities. Thus, even though at the Tevatron we start with the CP symmetric initial state, at the end of the day there can be slightly more -- than ++ dimuon final states. To study this effect, the D0 collaboration measured the asymmetry
The measurement is not as easy as it seems because there are pesky backgrounds that have to be carefully taken into account. The dominant background comes from ubiquitous kaons or pions that can sometimes be mistaken for muons. These particles may contribute to the asymmetry because the D0 detector itself violates CP: due to budget cuts the Tevatron abandoned construction of the D0 detector made of antimatter. In particular, the kaon K+ happens to travel further than K- in the detector material and may fake the positive value of asymmetry.
At the end of the day, after (hopefully) carefully subtracting the backgrounds, D0 quotes the measured asymmetry to be
Of course, it's too early to celebrate the downfall of the standard model, as in the past the bastard have recovered from similar blows. Yet there are reasons to get excited. The most important one is that the latest D0 result goes well in hand with the anomaly in the Bs system reported by the Tevatron 2 years ago. The asymmetry measured by D0 receives contributions from both Bs and Bd mesons. The Bd mesons are much better studied because they were produced by tons in BaBar and Belle, and to everyone's disappointment they were shown to behave according to the standard model predictions. However BaBar and Belle didn't produce too many Bs mesons (their beams were tuned to the Upsilon(4s) resonance which is a tad too light to decay into Bs mesons), and so the Bs sector can still hold surprises. Two years ago CDF and D0 measured CP violation in Bs decays into J/ψ φ, and they both saw a small, 2-sigma level discrepancy from the standard model. When these 2 results are combined with all other flavor physics data it was argued that the discrepancy becomes more than 3 sigma. The latest D0 results is another strong hint that something fishy is going on in the Bs sector.
Both the old and the new anomaly prompts introducing to the fundamental lagrangian a new effective four-fermion operator, ∼ (b s)2 + h.c., that contributes to the amplitude of Bs Bs oscillations. At this point we have no hints whatsoever what could be the source of this new operator, and the answer may even lie beyond the reach of the LHC. In any case, in the coming weeks theorists will derive this operator using extra dimensions, little Higgs, fat Higgs, unparticles, supersymmetry, old newspapers, golf balls, and tires. Yet the most important question is whether the asymmetry is real, and we're dying to hear from CDF and Belle... Looking forward to Session 06 of ICHEP, maybe we'll know more then :-)
Sunday, May 16, 2010
Dispensable Discoveries
Old data may well still hold in custody surprising things that we have yet to unearth: in the course of the last 70 years physicists have produced collisions between hadrons in a number of ways, inside detectors of all kinds. And while of course we cannot ex post improve the detecting devices with which those data were collected, we have in our hands today more computing power in a 30-bucks cell-phone than we had thirty years ago in a large experiment.
The corollary is that it may make sense to look back at old data, in search of surprises. But there is a catch: if you are going to do that, you have better try using the knowledge we have picked up in the meantime: if you do not, your work cannot be taken very seriously. The other catch is that it may require you to copy by hand data which is only archived in printed form, as is the case I am going to describe here!
The Narrow Resonance at "About 755 MeV"
The Cornell arxiv is, as always, the source. In a preprint appeared there a few days ago prof. Mario Gaspero, from University of Rome "La Sapienza" (a place ripe with highly distinguished physicists) claims the observation of a narrow resonance in antiproton-neutron collisions produced in 1970! Let us have a closer look.
The announcement appears to be not new -the paper is a writeup of a talk given in November 2009. However, I think I can be excused for having overlooked a talk on hadron spectroscopy held in Tallahassee. Less excusable is having missed basically the same information when it first appeared one year ago, in a previous preprint. Never mind -I can be a monster with a thousand eyes when I play chess, much less so when I browse preprints.
But what is this all about, after all ? Okay, I will describe it in short, but first let me quote from the Abstract:
"A narrow peak in the pi+/pi- mass distribution was seen by the Rome-Syracuse Collaboration in pbar n -> 2 pi+ 3 pi- annihilation at rest in 1970. It was ignored for 40 years."
I think that this remark sets the stage. Letting go with qualitative, grudge-bearing sentences like "it was ignored for 40 years" in the abstract of a single-authored paper is, in my humble opinion, an indicium that something is not kosher. From now on, the mind of a bastard like me opens a parallel processor during the reading: one which constantly tries to find other "crackpot" hints.
The abstract continues as follows:
"The reanalysis of this peak finds that it has the mass 757.4 [...] MeV and a width consistent with the experimental resolution. The evidence of the peak is 5.2 standard deviations. [...]"
Okay, there was an analysis, so this is a re-analysis. This to me means that the signal was not "ignored for 40 years". But it is interesting, and so the paper deserves to be read.
The data comes from a collaboration which studied the decay of the omega into two pions, using the Brookhaven National Laboratory's 30-inch bubble chamber. According to the author,
"in 1970, the Rome-Syracuse Collaboration (RSC) [...] found an unexpected result: the pi+ pi- mass distribution of 1496 annihilations at rest [...] had a narrow peak at about 755 MeV. This distribution is shown in Fig.1a. A chi^2 fit of this distribution found that the peak had a significance of about 4.5 standard deviations and a width lower than the experimental resolution. No relation was found between the pi+ pi- and other angular and mass distributions. These facts suggested to the RSC that the peak was generated by a fluctuation."
For those of you who are already feeling they are in the dark, let me explain in simple terms what this is about. First of all, while today we are used to create millions of proton collisions per second at ultra-TeV energies to understand the structure of matter at the shortest distance scales, and employ detectors that are allegedly the most complex creations of mankind ever, forty years ago we used collisions at energies of few GeV -the equivalent of a few proton masses- and inspected each collision within the volume of a vessel filled with liquid, by taking pictures at the trails of bubbles left by the charged particles produced in the collisions.
As for the "chisquare fit" and the "4.5 standard deviations": this is experimental physicist's jargon, to say how a mass distribution such as the one below may be approximated by a smooth curve, and suggest the presence of a significant feature. 4.5 standard deviations occur by chance as a random fluctuation of the background only a few times every million trials. Such a rarity is usually sufficient to claim that the feature is real, but this, crucially, assumes that one knows extremely well how the background is distributed.
Finally, the width. A short-lived particle has a finite width, but if the detector resolution is not good enough, the resonance curve in the mass distribution will look like a Gaussian shape with sigma equal to the resolution. If, however, a bump is found with a width significantly smaller than the experimental resolution -as appears the original observation in our case-, this cannot in any way be a resonance.
Professor Gaspero however feels compelled to investigate the effect in more depth. He continues by explaining that only by the early 1990s the properties of baryon annihilations started to get understood better, such that a prediction for the mass distribution -the background!- could be drawn on top of the 1970 data.
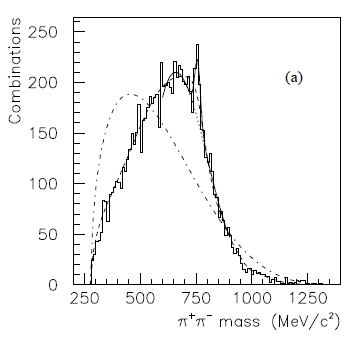
Anyway, let us follow the paper:
"A year after the communication of the preliminary results of this analysis, the OBELIX collaboration presented the preliminary results of the analysis [...]"
OBELIX was an experiment which studied a quite similar reaction; one that should have produced the same new particle.

"At that time, the absence of the peak in the OBELIX data confirmed the opinion that the RSC peak was a fluctuation".
So is this a fluctuation, after all ? One experiment sees a bump in an otherwise allegedly smooth distribution, this appears significant but not overly so, and another experiment does not see anything. Where is the crackpot index going to point at, in the end ?
The verdict
In the end, I believe professor Gaspero is innocent of the charges we have piled up against him. While the article may be written in a slightly non-conventional way, and while his fits are all less than convincing, he does have a point. The reason is to be found in another paper, which he finally mentions:
"Recently, Troyan et al. claimed to have observed several narrow pi+ pi- peaks in the reaction np -> np pi+ pi- with neutrons of 5.2 GeV/c. One of these peaks has a mass close to 755 MeV. The coincidence of the mass of the RSC peak with that of Troyan et al. suggested to reanalyse the RSC data."
Indeed, by checking the reference, one finds a nice mass distribution, where Troyan et al. imaginatively fit no less than 10 new resonant states of charged pion pairs! It seems far-fetched to believe these are all honest-to-god resonances, but in truth the bump sitting at 755 MeV is one of the most significant ones -it does look like a hadronic particle of small width.
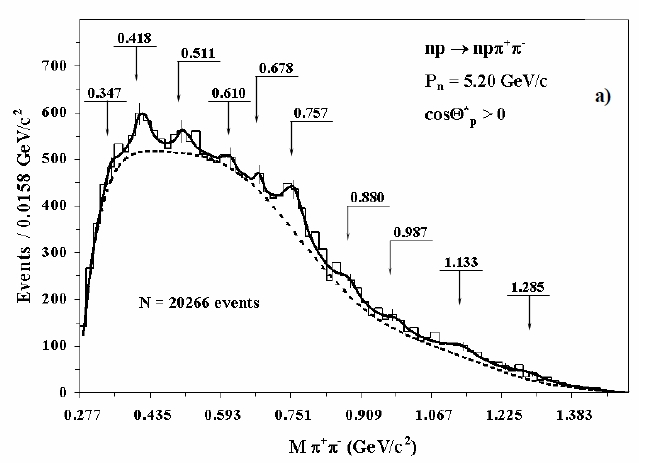
Above is the relevant figure. Admittedly, the "freedom fit" (one would usually call such smooth curves interpolating perfectly all the data points a "french fit", but let us this time to pay homage rather than mock our french friends) is a bit imaginative... But who knows: there are a lot of mysteries in low-energy quantum chromodynamics!
Gaspero in his paper tries to convince us that the excess observed by RSC at 755 MeV is significant enough to be considered a new particle. He estimates the significance of the observation in a way which is rather objectionable, but I do not wish to criticize such small prints. The particle may be there, or it may not. The problem, to me, is another. I sense, or imagine thanks to my fervid imagination, that this paper is written to get revenge rather than to claim a discovery which, leave alone belated, is not even a "first observation" -since if the particle is there, Troyan and collaborators are to be credited. Revenge is a strong word -let's say to have the final word on a controversy with colleagues who did not believe the new particle 40 years ago. Am I right ? I do not have a clue, and I do not wish to sound disrespectful to my colleague in Rome, so I will stop my conjectures here.
The problem is that the existence of these low-energy hadronic bound states does little to further our understanding of strong interactions, at least as long as they remain just mass peaks of unknown properties. Alas, we cannot predict them nor calculate their properties with the existing theoretical tools. If you let me take a radical stand, we need more theoretical ideas here! Lattice QCD may come to the rescue, but I believe there still are rough edges to smoothen there.
To conclude, I do not know if I learned something meaningful today, but I enjoyed reading this paper. And I imagine that in 2050 somebody from the OBELIX collaboration will come up with an explanation of why they did not, in fact, see the 755 MeV resonance... Stay tuned, but get yourself a comfortable chair!
Thursday, May 13, 2010
In and out
Saturday, May 8, 2010
In an exciting company!
So here I first of all wish to thank Marco Cirelli and the other organizers for realizing the value that physics blogs offer to the community.
And while I am at this, let me say that I have recently advocated a more official support of institutional web sites for the job of scientific blogs, and for their positive role for physics outreach and popularization of science. I did this at a conference, Comunicare Fisica 2010, held in Frascati one month ago. There, my ideas did not receive a lot of positive feedback. Nonetheless, being invited to be part of a free blogging effort under the sponsorship of an institution such as ICHEP is, in my opinion, worth much more than a thousand empty words -and tens of thousands had been spent on this subject after my suggestion in Frascati!
If you are interested, you may find more discussions on the above topic in my blog, as well as in the blog of Marco Delmastro -who is also going to contribute here- and Peppe Liberti. The latter two, however, only in Italian.
So I am exciting to see how this new endeavour develops! Stay tuned!
Sunday, May 2, 2010
A bit less than 3 months to ICHEP 2010
There are many reasons to look forward to this event. The LHC is working at full speed since a few weeks now and the collaborations are collecting precious data: expect exciting talks with long awaited results from them! But many other aspects of High Energy Physics will be covered in the 14 themes of the conference: sizable chunks of time will be devoted to Astroparticle Physics, Cosmology, Quantum Field Theory, Neutrinos, Flavor Physics, Computing and so on.
This year will also be an important date to shape up the future of HEP. Several major projects will reach milestones and decisions will be taken. Many of these issues will be discussed in dedicated sessions of the Conference.
After so many successful ICHEP conferences, let's look forward to another unforgettable one!
This blog hosts the contributions of a distinguished group of blogging physicists who have accepted to share their interests and enthusiasm in the occasion of the Conference. It will serve as a communication tool both towards the conference participants, the scientific community and the interested public in general. Thanks to all those who have agreed to take part in this adventure! If you are interested in joining us, please contact the ICHEP Organizing Committee.